Abstract
A well-functioning chain of survival is critical for good outcomes following out-of-hospital cardiac arrest, a major public health concern in Singapore. While the percentage of survivors to hospital admission has increased over the years, the percentage of survivors to hospital discharge and the number of patients with good neurological recovery can be greatly improved. This underscores the urgent need to focus on ‘post-cardiac arrest care’, the fifth link in the chain of survival, to improve the outcomes of patients who are admitted to the intensive care unit (ICU) after return of spontaneous circulation. This review builds on earlier recommendations of the Singapore National Targeted Temperature Management Workgroup in 2017 to provide a focused update on post-cardiac arrest management and a practical guide for physicians managing resuscitated patients with cardiac arrest in the ICU.
CASE VIGNETTE (PART 1)
Ms T was a 28-year-old woman with no significant past medical history. She experienced chest pain and collapsed at her workplace at 1120 hours. On arrival of the paramedics, she was in pulseless electrical activity. Adrenaline was administered and she developed ventricular fibrillation (VF). Upon defibrillation, she had transient return of spontaneous circulation (ROSC). On arrival at the emergency department, the patient had recurrent VF, which required further defibrillations and adrenaline. Amiodarone was also administered. There was still no sustained ROSC at 1230 hours (which was after 23 minutes of ‘no flow’ and 47 minutes of ‘low flow’ time). At that time, arterial blood gas analysis revealed pH 6.89, partial pressure of carbon dioxide in arterial blood (PaCO2) 34 mmHg, partial pressure of oxygen in arterial blood (PaO2) 117 mmHg, bicarbonate (HCO3) 7 mmol/L, base excess −27 mmol/L and arterial oxygen saturation (SaO2) 94%. The emergency physician and cardiologist made a joint decision to send the patient to the cardiac catheterisation laboratory on a mechanical chest compression device. Emergency coronary angiogram revealed a spontaneous left main stem dissection, for which percutaneous coronary intervention (PCI) was performed with implantation of a drug-eluting stent. The patient achieved sustained ROSC shortly after PCI with a total ‘downtime’ of 112 minutes and was transferred to the cardiac intensive care unit (ICU) for further management.
INTRODUCTION
Out-of-hospital cardiac arrest (OHCA) is a major public health concern in Singapore. Based on statistics from the Ministry of Health, Singapore, the incidence of cardiac arrest increased from 26.5 to 52.7 patients per 100,000 population from 2011 to 2018.(1) A well-functioning chain of survival is critical for good outcomes following OHCA. Improvements in pre-hospital interventions, such as bystander cardiopulmonary resuscitation, wider availability and usage of automated external defibrillators and technologies such as the myResponder App, have increased the percentage of survivors to hospital admission from 18.2% to 18.5%. However, after admission to the ICU, survival to hospital discharge remains low at only 5.9%. More importantly, among survivors, there is often significant neurological damage that imposes disabling immediate and long-term consequences on the patients, their families and the broader community. Of note, only 4.0% of patients with cardiac arrest in Singapore survive with moderately good to good neurological function. This underscores the importance of focusing on ‘post-cardiac arrest care’, the fifth link in the chain of survival, to improve the outcomes of patients who are admitted to the ICU after ROSC.
Achieving ROSC is only the first step towards the goal of complete recovery after cardiac arrest. The complex pathophysiological processes that occur following global ischaemia during cardiac arrest and the subsequent reperfusion response during and after resuscitation are termed the ‘Post-Cardiac Arrest Syndrome’ (PCAS).(2) PCAS comprises four components: post-cardiac arrest brain injury; post-cardiac arrest myocardial dysfunction; systemic ischaemic-reperfusion syndrome; and persistence of precipitating pathology, with brain injury accounting for most of the morbidity and mortality after ROSC. The optimal care of PCAS requires coordinated management of multiple organ systems, including targeted temperature management (TTM). Depending on the cause of collapse and severity of PCAS, many patients will require multiorgan support, and the treatments that they receive during the post-resuscitation period significantly influence overall outcomes and, in particular, neurological recovery.(3) Therefore, treatments to optimise ventilatory, haemodynamic and metabolic variables, together with TTM, should be initiated as soon as possible. This review builds on earlier recommendations of the Singapore National TTM Workgroup in 2017,(4) as well as the International Liaison Committee on Resuscitation (ILCOR) 2020 guidelines,(5) to provide a focused update on post-cardiac arrest management (the ‘A to I’ approach) and a practical guide for physicians managing resuscitated patients with cardiac arrest in the ICU (
Table I
Summary of recommendations for post-cardiac arrest management.
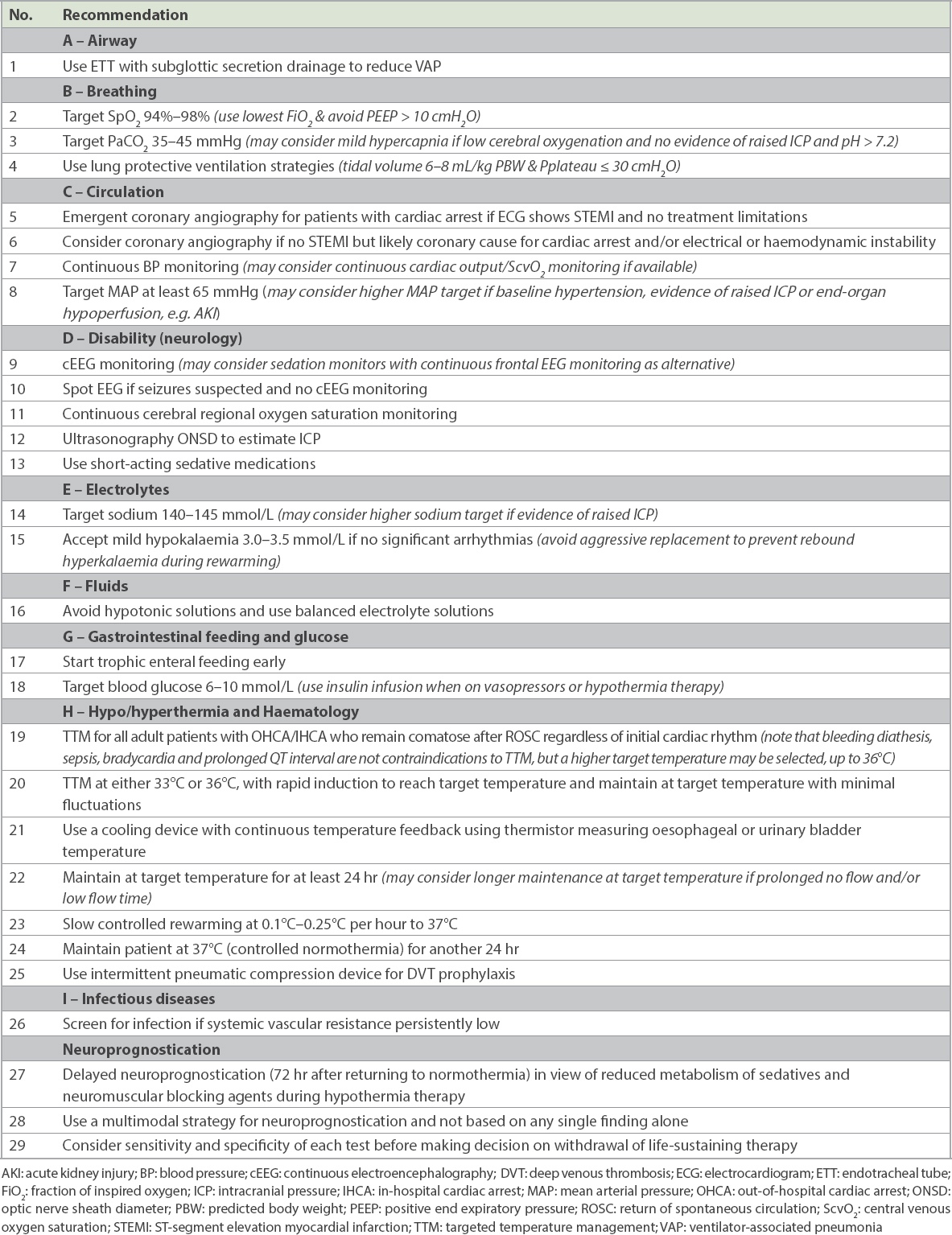
Fig. 1
Management of post-cardiac arrest syndrome: the ‘A to I’ approach. DVT: deep venous thrombosis; EEG: electroencephalography; ETT: endotracheal tube; ICP: intracranial pressure; KIV: keep in view; MAP: mean arterial pressure; PEEP: positive end expiratory pressure; ScvO2: central venous oxygen saturation; US: ultrasonography; VAP: ventilator-associated pneumonia [adapted with permission from Ng S and Chia YW].(30)
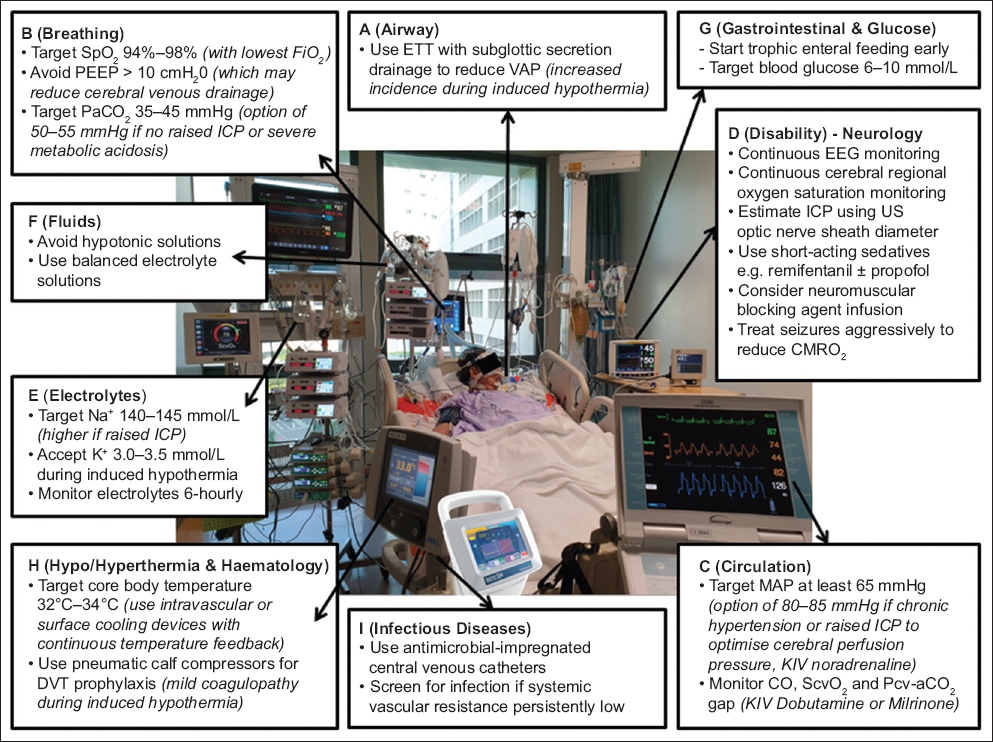
A – AIRWAY
Patients who only have a brief period of cardiac arrest and respond immediately to resuscitation may achieve a return of normal cerebral function and may not require intubation if they are able to maintain their airway. However, if there is any doubt about the ability to protect airway such as a depressed conscious level, tracheal intubation and mechanical ventilation should be instituted. It is reasonable to consider using a tracheal tube with subglottic secretion drainage to reduce ventilator-associated pneumonia, which has a higher incidence during TTM.(6,7)
B – BREATHING
Oxygenation
Hypoxaemia (PaO2 < 60 mmHg or PaO2/fraction of inspired oxygen [FiO2] ratio < 300) increases the likelihood of further cardiac arrest and contributes to secondary brain injury. Conversely, hyperoxaemia (PaO2 ≥ 300 mmHg) has the potential to cause oxidative stress, worsening neurological injury and increasing mortality.(8,9) The recommendation, therefore, is to target blood oxygen saturation (SpO2) of 94%–98%. We should also avoid using a high level of positive end expiratory pressure (PEEP; e.g. > 10 cmH2O), as high intra-thoracic pressures may reduce cerebral venous drainage, increasing cerebral blood volume and intracranial pressure (ICP). Thereafter, FiO2 should be titrated to achieve oxygenation targets.
Ventilation
Hypocapnia causes cerebral vasoconstriction and reduces cerebral blood flow, contributing to poorer neurological outcomes. Conversely, pilot studies have shown that mild hypercapnia is associated with better neurological prognosis in post-cardiac arrest patients.(10-13) Until definitive studies supporting mild hypercapnia are reported, the current recommendation is to aim for normocapnia, i.e. target PaCO2 35–45 mmHg. It may be reasonable to consider targeted therapeutic mild hypercapnia, i.e. PaCO2 50–55 mmHg, if there is evidence of low cerebral oxygenation and no contraindications to mild hypercapnia such as raised ICP or severe metabolic acidosis. Although the in vivo PaCO2 decreases by 2 mmHg for each degree Celsius below 37°C, there is currently no recommendation for temperature correction of CO2. The use of end tidal carbon dioxide (ETCO2) for continuous monitoring is invaluable and the PaCO2-PETCO2 gradient should be determined daily. Both hypothermia and the use of neuromuscular blocking agents can reduce CO2 production and increase the risk of hypocapnia.(14) Routine lung protective ventilation strategies, i.e. tidal volume 6–8 mL/kg, predicted body weight and maintaining plateau pressure ≤ 30 cmH2O, should be employed to reduce the incidence of ventilator-induced lung injury. Chest radiography should be performed to confirm the positions of tracheal tube, gastric tube and central venous catheter, and to detect pulmonary oedema, pneumonia and complications arising from resuscitation (e.g. rib fractures).
C – CIRCULATION
Coronary angiography
Acute coronary syndrome is a frequent cause of OHCA, and 59%–71% of patients with OHCA without an obvious non-cardiac cause have an acute coronary lesion found on coronary angiography.(15) As such, urgent coronary angiography should be performed in patients after ROSC if the electrocardiogram (ECG) shows ST-segment elevation (Class I recommendation by both American Heart Association [AHA] and European Society of Cardiology [ESC]).(16-19) For patients with no ST-segment elevation, it is reasonable to consider emergent coronary angiography if they have a high probability of a coronary cause for the arrest and/or have ongoing electrical or haemodynamic instability (Class IIa recommendation by AHA and ESC).(17-20)
Haemodynamic monitoring
Treatment should be guided by blood pressure (BP), cardiac output, central venous oxygen saturation (ScvO2), urine output and lactate clearance. An arterial cannula should be inserted for continuous BP monitoring. As cardiac function is often impaired after cardiac arrest, it is also reasonable to consider continuous cardiac output and continuous ScvO2 monitoring. Serial focused ultrasonography for fluid responsiveness and echo-Doppler techniques to monitor stroke volume may also be performed.
Haemodynamic targets
Although the optimal target for mean arterial pressure (MAP) remains debatable, it is recommended to consider the patient’s baseline BP and any evidence of raised ICP or acute kidney injury. In the absence of invasive ICP monitoring, an ultrasonography assessment of the optic nerve sheath diameter (ONSD) > 5 mm correlates well with an ICP > 20 cmH2O.(21) During PCAS, cerebral autoregulation is impaired and cerebral blood flow varies directly with cerebral perfusion pressure.(22) The recommended MAP target is at least 65 mmHg, and a higher MAP target (e.g. 80–85 mmHg) may be reasonable if the patient has chronic hypertension, or evidence of raised ICP or end-organ hypoperfusion.
Bradycardia
Bradycardia is common during induced mild hypothermia. It usually does not result in haemodynamic compromise and may be associated with better neurological outcomes.(23) Therefore, there is no need to intervene if the patient develops sinus bradycardia with a heart rate of 30–40/min, if the BP, ScvO2 and lactate clearance are adequate. However, if there is advanced atrioventricular block, the target temperature can be increased slightly, up to 36°C.
Haemodynamic support
Post-resuscitation myocardial dysfunction causes haemodynamic instability, manifesting as hypotension, low cardiac output and arrhythmias.(24) Myocardial dysfunction often requires inotropic support, with dobutamine having the most evidence for use.(25) However, the systemic inflammatory response syndrome (SIRS) that occurs in PCAS also causes vasodilation and, sometimes, vasoplegia. If vasoplegia predominates, it is recommended to start noradrenaline first to achieve haemodynamic targets and also because it is less arrhythmogenic.(26) However, if up-titration of noradrenaline reduces stroke volume or ScvO2 (suggesting an excessive increase in left ventricular afterload causing a drop in cardiac output), it may be useful to add on a low-dose inotrope (e.g. dobutamine 3–5 mcg/kg/min). Noradrenaline, with or without an inotrope, is usually the most effective therapeutic regime.
D – DISABILITY (NEUROLOGY)
Indications for computed tomography
In the absence of preceding cardiac symptoms and ischaemic ECG changes, and/or in the presence of symptoms or signs suggestive of a neurological cause (headache, seizures, focal neurological deficits) for the cardiac arrest, computed tomography (CT) of the head should be considered.(27) Similarly, if a respiratory cause (dyspnoea, hypoxia in patients with known respiratory disease) is suspected, CT of the thorax should be considered.
Cerebral monitoring
Seizures are common after anoxic brain injury and occur in approximately one-third of patients who remain comatose after ROSC. Therefore, patients should be on continuous electroencephalography (cEEG) monitoring. If cEEG is not available, limited evidence suggests that sedation monitors may be helpful, as they provide four channels of continuous frontal EEG monitoring. A spot EEG should be obtained whenever there is clinical suspicion of seizures if patient is not already on EEG monitoring. In addition, cerebral blood flow and cerebral tissue oxygenation may be indirectly monitored using near-infrared spectroscopy to measure cerebral regional oxygen saturation (rSO2).(28-30) The use of cerebral rSO2 is useful to guide management. For example, up-titration of noradrenaline to achieve a particular MAP target may result in decreased cerebral blood flow from cerebral vasoconstriction and/or a drop in stroke volume from increased left ventricular afterload. The decrease in cerebral blood flow will manifest as a drop in cerebral rSO2. A drop in rSO2 can also be an early warning of hypocapnia from hyperventilation to reduce ICP or after the start of neuromuscular blocking agent for shivering during induction of hypothermia.(14,28)
Sedation
Adequate sedation reduces oxygen consumption and improves the balance between oxygen supply and demand. Sedation also helps to reduce the incidence of shivering during induced hypothermia. It is also important to start sedation if neuromuscular blocking agents are used, to prevent awareness during paralysis. Although there is no data to support whether the choice of agents affects outcome, it is recommended to use short-acting drugs (e.g. remifentanil and propofol), as they allow more reliable and earlier neurological assessment.(31) This is especially important, as the metabolism of sedatives and neuromuscular blocking agents is reduced with hypothermia.
Paralysis
Limited data suggests that continuous infusion of neuromuscular blocking agents is associated with decreased mortality in PCAS.(32) However, the use of neuromuscular blocking agents interferes with clinical examination and masks seizures. Therefore, cEEG or a sedation monitor with continuous frontal EEG monitoring should be utilised whenever patients are on paralytics.(33)
Seizures
Myoclonus is the most common seizure and occurs in 18%–25% of patients, the remainder being focal or generalised tonic-clonic seizures.(34) Seizures increase the cerebral metabolic rate of oxygen (CMRO2) and can potentially result in secondary brain injury. They should be treated aggressively, and the recommended options are levetiracetam and sodium valproate, as they have less adverse cardiac effects.
E – ELECTROLYTES
Regarding serum electrolytes, aim for normal sodium level (e.g. 140–145 mmol/L), but if ICP is raised, the target can be increased (e.g. to 150–155 mmol/L). Mild hypokalaemia during hypothermia is common because of cold diuresis and transcellular shift. Accept mild hypokalaemia (e.g. 3.0–3.5 mmol/L) if there are no significant arrhythmias, and avoid aggressive replacement to prevent rebound hyperkalaemia during subsequent rewarming.
F – FLUIDS
Choice of fluids
Cerebral oedema may occur transiently after ROSC, but it is rarely associated with a clinically relevant increase in ICP. Nonetheless, it is important to avoid hypotonic solutions, which may worsen brain swelling. Balanced electrolyte solutions such as lactated Ringer’s Solution and Plasma-Lyte A are recommended. These potassium-containing fluids may also help to reduce the hypokalaemia commonly encountered during hypothermia.
G – GASTROINTESTINAL FEEDING AND GLUCOSE
Early enteral feeding is recommended as per standard ICU practice to reduce infectious complications. However, feeding should be started at low rates (trophic feeding), as hypothermia may lead to gastroparesis and prolonged intestinal transit time. There could be high gastric residual volume requiring the use of prokinetic agents. A combination of metoclopramide and erythromycin has been shown to have synergistic effects in reducing gastric residual volume.(35) Monitor for QT interval prolongation if the patient is on both erythromycin and hypothermia therapy.
Both low and high blood glucose levels have adverse effects on the neurological prognosis.(36) Hypothermia is associated with both hyperglycaemia (because of reduced insulin sensitivity) and increased blood glucose variability, both of which are associated with increased mortality and unfavourable neurological outcomes after cardiac arrest. Based on the available data, the recommendation is to target normoglycaemia (e.g. blood glucose 6–10 mmol/L). Use intravenous insulin infusion, rather than subcutaneous insulin, to control blood glucose levels when the patient is on vasopressors and/or hypothermia therapy, as subcutaneous absorption may be erratic.
H – HYPOTHERMIA/HYPERTHERMIA
TTM includes both targeted hypothermia (targeting core body temperature 32°C–34°C) and targeted normothermia (targeting core body temperature 35°C–37°C). Guidelines advocate TTM for all adult patients with OHCA and in-hospital cardiac arrest (IHCA) who remain comatose after ROSC regardless of initial cardiac rhythm.(18,19) A target temperature of 32°C–36°C should be selected, achieved and maintained consistently for at least 24 hours. Data indicates that mild hypothermia is neuroprotective and improves outcomes after ROSC.(37-39) Cooling suppresses pathways that lead to apoptosis, decreases CMRO2 by 6% with every degree Celsius reduction in core body temperature, and reduces the release of excitatory amino acids and free radicals. Conversely, several studies have documented an association between post-cardiac arrest pyrexia and poor neurological outcomes.(40) Therefore, it is reasonable to treat hyperthermia occurring after cardiac arrest with antipyretics and consider the use of active cooling.
The 2013 TTM Trial randomised 950 patients with OHCA to TTM at 33°C vs. 36°C, and the results showed no difference in all-cause mortality or neurological outcomes at the 180-day follow-up.(41) Unfortunately, this led to some centres misinterpreting the trial results and abandoning TTM use altogether. It is important to note that the TTM Trial had two active treatment arms (i.e. TTM at 33°C vs. TTM at 36°C). It was not a trial of TTM versus no TTM. Active temperature management was also advocated for patients randomised to the 36°C arm. Centres that have changed their target temperature from 33°C to 36°C have shown that maintenance of target temperature at 36°C is technically more challenging compared to 33°C (time in target temperature 50% vs. 87%), as is the avoidance of pyrexia (incidence of fever 19% vs. 0%), with a trend towards increased mortality.(42) Therefore, it is important for ICUs adopting a strategy of TTM at 36°C to be aware that patients on targeted normothermia still require active temperature management, including the use of sedatives, neuromuscular blocking agents and cooling devices, to minimise fluctuations in temperature and prevent fever.
In line with international guidelines, the Singapore National TTM Workgroup recommends TTM for all adult patients with ROSC after OHCA or IHCA who are unable to obey commands (Glasgow motor score < 6), regardless of initial cardiac rhythm (i.e. VF, ventricular tachycardia, pulseless electrical activity or asystole).(18,19,43)
Exclusion criteria for TTM: (a) patients deemed unsuitable for further resuscitation; and/or (b) patients with baseline Cerebral Performance Category 3 or 4. (Note that bleeding diathesis, sepsis, bradycardia and prolonged QT interval are not contraindications to TTM. Cardiac arrest patients with these conditions will still benefit from TTM, but a higher target temperature may be selected, up to 36°C.)
We recommend targeting a temperature of 33°C for PCAS (range 32°C–34°C). However, for patients who are intolerant of 33°C (e.g. those with bleeding diathesis, significant bradycardia or marked QT interval prolongation), it is reasonable to aim for a higher target temperature of up to 36°C. A cooling method with effective temperature monitoring that minimises temperature fluctuations is preferred. This is best achieved with internal or external cooling devices that have continuous temperature feedback to achieve a set target temperature, using thermistors placed in the oesophagus or urinary bladder to monitor the core body temperature.(44) Temperature should be monitored continuously and recorded at least hourly in the clinical charts. Endovascular cooling catheters and gel-coated adhesive cooling pads provide more rapid hypothermia induction and more effective temperature maintenance with minimal fluctuations compared to water-circulating cooling blankets.(45)
Induction phase
- If target temperature is 33°C and the patient’s temperature is below 33°C, allow passive (not active) rewarming to 33°C, and then start endovascular or surface cooling to maintain the temperature at 33°C.
- If target temperature is 33°C and the patient’s temperature is above 33°C, start endovascular or surface cooling to reach the target temperature of 33°C. Avoid rapid infusion of large volumes of cold fluids, which have been shown to cause pulmonary oedema and recurrent cardiac arrest.
- If target temperature is 36°C and the patient’s temperature is below 36°C, allow passive (not active) rewarming to 36°C, and then start endovascular or surface cooling to maintain the temperature at 36°C.
- If target temperature is 36°C and the patient’s temperature is above 36°C, start endovascular or surface cooling to reach the target temperature of 36°C.
Maintenance phase
Maintain the patient at the target temperature for at least 24 hours after reaching the target temperature. It may be reasonable to consider maintaining a longer duration at the target temperature for patients who have a longer duration of no flow and/or low flow time.(46) Shivering increases metabolic rate and causes heat production. The occurrence of shivering in patients who undergo mild hypothermia is associated with better neurological outcomes as it is a sign of normal physiological response. Management of shivering includes: (a) skin counter-warming; (b) intravenous magnesium; (c) increasing sedation; and (d) starting paralytics (put on cEEG or sedation monitoring, as seizures will be masked).
Rewarming phase
Rewarm the patient very gradually (e.g. at 0.1°C–0.25°C per hour) until the temperature reaches 37°C, and maintain the temperature at 37°C (controlled normothermia) for another 24 hours. The development of hyperthermia after induced mild hypothermia (i.e. rebound hyperthermia) is associated with increased mortality and worse neurological outcomes.(47,48) Therefore, slow, controlled rewarming at 0.1°C–0.25°C per hour is recommended. Other effects during rewarming include: (a) increased oxygen consumption; (b) increased CO2 production from increased metabolic activities (may need to increase minute volume to maintain normocapnia); (c) hypotension from vasodilation (do not wean off noradrenaline too early); (d) tachyarrhythmias, which may cause myocardial ischaemia; (e) cerebral desaturation from increased CMRO2 (continue to monitor cerebral rSO2); (f) seizures from lowering in seizure threshold (continue to monitor EEG); (g) rebound hyperkalaemia from transcellular shift (keep potassium low-normal before rewarming); and (h) hypoglycaemia from increased insulin sensitivity (may need to reduce insulin infusion).
H – HAEMATOLOGY
Hypothermia results in mild coagulopathy. However, there is usually no clinically significant bleeding and, therefore, the use of antiplatelets and anticoagulants is not contraindicated. However, if there is bleeding, a higher target temperature may be selected, up to 36°C. Intermittent pneumatic compression device should be applied for deep venous thrombosis prophylaxis.
I – INFECTIOUS DISEASES
There is a higher incidence of lower respiratory tract infections with hypothermia therapy because of mild immune paresis.(6) However, there is no current recommendation for prophylactic antibiotics.(49) The SIRS response in PCAS should resolve in the first 24–48 hours after cardiac arrest. If systemic vascular resistance remains persistently low, screen for sepsis and check infective markers, as the patient will not mount a fever response during TTM.
NEUROPROGNOSTICATION
A multimodal strategy, comprising clinical examination, somatosensory-evoked potentials, EEG, serum biomarkers of neuronal damage and cerebral imaging, should be used for neuroprognostication.(50) The clearance of sedative drugs and neuromuscular blocking agents can be reduced by up to 30% at a core body temperature of 34°C. Therefore, in patients who are cooled to 32°C–34°C, neuroprognostication using clinical examination should be delayed until 72 hours after returning to normothermia.(18) Accurate neurological prognostication in brain-injured cardiac arrest survivors is crucial to ensure that patients with potential for meaningful recovery are not destined for certain poor outcomes because of withdrawal of life-sustaining therapy.
CONCLUSION
Post-cardiac arrest care is a critical link in the chain of survival and demands a comprehensive, structured, multisystem approach to improve neurologically intact survival. The quality of post-resuscitation ICU care has a major influence on the final clinical outcome. If a patient with cardiac arrest is ‘fortunate’ enough to be successfully resuscitated, he or she deserves the best chance for a good long-term prognosis.
CASE VIGNETTE (PART 2)
The patient was ventilated with a lung protective strategy with low PEEP of 5 cmH2O, accepting SpO2 95%. Her haemodynamics were supported with intra-aortic balloon pump counterpulsation, noradrenaline and vasopressin. Ultrasonography ONSD was 7 mm, indicating a raised ICP of > 20 cmH2O. Therefore, the MAP target was set at 80–85 mmHg to optimise cerebral perfusion pressure. Osmotherapy with hypertonic saline was also started to lower ICP, aiming for a sodium target of 150–155 mmol/L. PaCO2 was lowered gradually from 55 mmHg, with continuous cerebral rSO2 monitoring. It was noted that cerebral rSO2 plummeted from 48% to 41% when PaCO2 reached 37 mmHg. Therefore, PaCO2 target was set at 40–45 mmHg. Hypercapnia was avoided because of raised ICP.
Continuous cardiac output monitoring revealed a cardiac index of 2.3 L/min/m2, a mixed venous oxygen saturation (SvO2) of 67% with Pmv-aCO2 gap of 10 mmHg and arterial lactate > 10.8 mmol/L, suggesting a low flow state. Therefore, low-dose dobutamine was added, which led to an increase in cardiac index to 3.0 L/min/m2, an increase in SvO2 to 76%, a reduction in Pmv-aCO2 gap to 5 mmHg and a drop in lactate to 4.5 mmol/L. Cerebral rSO2 also increased to 59% after dobutamine was started, reflecting an increase in cerebral blood flow.
On arrival in the cardiac ICU, the patient was commenced on intravascular temperature management, reaching a target temperature of 33°C from 35.8°C within 45 minutes. Repeat ultrasonography ONSD at 24 hours revealed that the ICP was still elevated and, therefore, rewarming was not attempted. She was maintained at target temperature for about 72 hours and gradually rewarmed when ONSD had normalised. She was rewarmed at 0.1°C per hour to 37°C (over 40 hours) and maintained at 37°C (controlled normothermia) for another 24 hours.
The patient woke up after reaching normothermia when atracurium and remifentanil were weaned off. She was able to obey commands and recognise her family members. She was subsequently extubated and transferred to the general ward. The patient was in Cerebral Performance Category 1 with a normal neurocognitive assessment before discharge.