Abstract
INTRODUCTION
This study aimed to investigate the therapeutic response to injected human umbilical cord blood mesenchymal stem cells (UCBMSCs) among albino rats with streptozotocin (STZ)-induced diabetes mellitus.
METHODS
Control group (GI; n = 25) rats were fed with standard rat diet. Rats with STZ-induced diabetes mellitus without (GII; n = 25) and with (GIII; n = 25) differentiated human UCBMSCs implantation were the test groups. Rats were sacrificed in Week 11 following implantation. Liver biopsies were sectioned and stained in order to highlight both the presence and function of impregnated cells in the liver tissue.
RESULTS
Haematoxylin and eosin-stained sections in GI and GII rats showed normal liver architecture while GIII rats showed presence of cell clusters inside the liver tissue and around the central veins. Cell clusters with blue cytoplasm were present in sections in GIII rats but absent in GI and GII rats, indicating the presence of injected differentiated human UCBMSCs. The anti-human insulin immunostaining of GIII rats showed clusters of cells within the liver parenchyma and around central veins, indicating that these cells were active and secreting insulin.
CONCLUSION
UCBMSCs are proficient in differentiating into insulin-producing cells in vivo under specific conditions and, when transplanted into the liver of albino rats with STZ-induced diabetes mellitus, were able to secrete insulin and partially control the status of diabetes mellitus in rats.
INTRODUCTION
Diabetes mellitus is one of the most prevalent chronic diseases affecting people worldwide. Reports by the International Diabetes Federation in 2011 stated that the prevalence of Type II diabetes mellitus (TIID) had already reached 366 million in 2011 and was expected to touch alarming levels at 552 million by 2030.(1) This increase is thought to be attributed to various factors, such as rapid urbanisation, rise in population, ageing and decreased physical activity coupled with the high prevalence of obesity.(1) There is also an increased concern over the rising tide of Type I diabetes mellitus (TID) among children. A recent report showed a 70% increase in TID incidence among children aged less than 15 years and twice this number in children aged less than five years.(2) According to reports of the American Diabetes Association in 2000, the incidence of TIID, which was previously uncommon among children, has also shown a rapid increase of 15%–45% and is therefore considered to be a global epidemic.(3) Moreover, a worldwide estimation in 2010 showed that diabetes mellitus accounted for about 12% (USD 376 billion) of healthcare expenditures and is believed to hit USD 490 billion by 2030.(4) This continuous rise in the incidence of diabetes mellitus poses a serious global health threat and calls for further investigations to find a cure.
In the past decade, there have been great advances in the treatment of TID, with revolutionary success in pancreatic islet cell transplantation. Nevertheless, due to alloimmune graft rejection and the inadequate supply of organ donors, this type of treatment still caters only to a small number of patients.(5) The search for new therapeutic strategies is thus still ongoing.
Stem cell-based therapies and gene therapies are currently considered by many researchers as an alternate way of treating diabetes mellitus. Mesenchymal stem cells (MSCs), which are known for their potential to differentiate into multiple cell lineages, are now considered an ideal tool for developing new cell-based therapeutic approaches.(6) Currently, bone marrow is the main source of MSCs when compared to various other tissues, such as adipose tissue, human umbilical cord blood, dental pulp and menstrual blood.(7-10) However, due to the less invasive nature of the procedure, easy availability, weaker immunogenicity and various other notable characteristics, human umbilical cord blood MSCs (UCBMSCs) appear to be a better substitute to bone marrow-derived MSCs.(11)
The present study aimed at investigating and evaluating the potential therapeutic role of transplanted differentiated human UCBMSCs in albino rats with streptozotocin (STZ)-induced diabetes mellitus.
METHODS
75 adult albino rats were purchased from Mansoura Faculty of Pharmacy and Medical Experimental Research Center of Mansoura University, Mansoura, Egypt. All rats were housed in climate-controlled rooms, with pathogen-free conditions, having open access to pellet food and water. All protocols for animal experimentation followed the guidelines of the National Institutes of Health, USA, and were reviewed and approved by the committee of animal experimentation at Mansoura University, Egypt.
The animals were randomly divided into three groups: control group (GI, n = 25); diabetes mellitus group without stem cell transplantation (GII, n = 25); and diabetes mellitus group with differentiated MSC transplantation (GIII, n = 25).
All animals were sacrificed ten weeks after transplantation of differentiated MSC, and were subjected to histopathological and immunohistochemical studies.
For STZ-induced diabetes mellitus, STZ (Sigma-Aldrich, St. Louis, MO, USA) was mixed in sodium citrate buffer (pH 4.5) and administered intraperitoneally within 15 minutes of preparation. The rats were fasted for 12 hours prior to the experiment following which a daily dose of STZ (dose 40 mg/kg) was introduced intraperitoneally under light ether anaesthesia.
Confirmation of the status of diabetes mellitus was done by determining blood glucose levels (Accu-Chek® Active; Roche Diagnostic GmbH, Mannheim, Germany) from the third day after the first shot of STZ. Animals were confirmed to have diabetes mellitus when fasting blood glucose was over 13.9 mmol/L (or 250 mg/dL) for two successive days.
For the preparation of human UCBMSCs, umbilical cord blood samples from full-term deliveries were collected from the Obstetrics and Gynecology departments of Mansoura University and Cairo University, Egypt. Written informed consent to use the cord blood for research was taken from all patients. All procedures were performed under highly aseptic conditions. Following the delivery of the baby, the cord was double clamped. Blood was obtained from the maternal end of the cord by inserting a needle into the umbilical vein above the clamp and thereafter drained via gravity into a sterile collection bag containing 2 mL of heparin. All samples were then immediately separated for culturing. The study was approved by the ethics committees at Mansoura University and Cairo University.
Human umbilical cord blood mononuclear cells were isolated at room temperature by density gradient centrifugation at 1,800 rotations per minute (rpm) for 20 minutes using Ficoll solution (Biochrom Ltd, Cambourne, Cambridge, UK). Following centrifugation, the pellets containing mononuclear cells were washed and resuspended in Dulbecco’s Modified Eagle’s Medium (DMEM) and further centrifuged at 1,800 rpm for 20 minutes. The precipitated cell pellets in tubes containing 1 mL DMEM culture media were checked for viability using trypan blue 1% dye. Estimation of the viable cells was performed using haemocytometer. The mononuclear cells were resuspended in 20 mL of low glucose DMEM, supplemented with a cocktail of 10% fetal calf serum, penicillin (100 U/mL/L), amphotericin B (5 µg/mL) and streptomycin (100 µg/mL). They were later transferred into T25 tissue culture flasks and cultured under humidified atmosphere containing 5% carbon dioxide and 95% air at 37°C for 24 hours. Media was changed every three days until the cells attained 80%–90% monolayer confluence. Successively, the adherent cells were detached for subculturing and further identification using 0.25% trypsin-EDTA (ethylenediaminetetraacetic acid; 1 mM) and incubated at 37°C. The flask was gently rocked to enable detachment of cells and was monitored periodically through a microscope. Further neutralising of trypsin-EDTA to displace the cells was done by adding prewarmed culture media (5 mL). It was then centrifuged at 1,500 rpm for five minutes, and the cell pellet was collected and resuspended into new media. These cells were counted using a haemocytometer and tested for viability.
Detecting and quantifying the expression of CD34, CD44 (which are haematopoietic stem/progenitor cell markers) and intracellular marker molecules (Oct 3/4) were done using flow cytometry. After labelling the monoclonal antibodies with fluorescein isothiocynanate, cells were incubated at room temperature for 20 minutes against CD34/CD44 (Coulter Immunotech, Beckman Coulter, Marseille, France) and analysed by FACSCalibur flow cytometer (Immunotech SAS, Beckman Coulter, Marseille, France). Further incubation of the cells for 30 minutes followed by phycoerythrin (PE)-conjugated anti-human/mouse Oct 3/4 monoclonal antibodies were done after fixation and permeabilisation, and these were analysed using FACSCalibar flow cytometer.
For the in vitro differentiation of UCBMSCs into functional islet pancreatic cells, the undifferentiated UCBMSCs with 80% confluence were cultured for one week (at 37°C, 5% carbon dioxide) in serum-free, glucose-rich DMEM (25 mM/L) (Sigma-Aldrich Chemie GmbH, Germany); 10%; fetal bovine serum (Lonza Bioproducts, Verviers, Belgium); 10 nM/L Exendin-4 (Sigma Aldrich, Sigma-Aldrich Chemie GmbH); 0.5% amphotericin B (Thermofisher Scientific, Paisley, UK); 1% penicillin and streptomycin (Thermofisher Scientific).
In vitro assessment and confirmation of differentiation of UCBMSCs into islet pancreatic cells prior to transplantation into the rat liver was performed using various methods: (a) for morphological changes, clusters of islet-like cells were identified after seven days of culturing; (b) test for viability of cells was done using trypan blue; (c) for detection of insulin gene expression by reverse transcription polymerase chain reaction (RT-PCR), gene expression profiles of insulin for both undifferentiated and differentiated UCBMSCs were assessed using RT-PCR; (d) for analysis of insulin expression by flow cytometry, differentiated UCBMSCs were tested for differentiation by quantitating cells expressing cytoplasmic insulin through flow cytometric analysis using anti-human/bovine/mouse insulin-allophycocyanin monoclonal antibody (R&D Systems, Canada). Measurement of positive insulin rate was done by FACSCalibur (BD Biosciences, San Jose, CA, USA). Isotype-matched antibodies served as controls for autofluorescence; and (e) for insulin detection assay, to estimate total insulin levels, the total cell protein content was tested using the BCA Protein Assay Kit (Cambridge, MA, USA). Measurement of secreted insulin was performed with the chemiluminescence immunoassay system ADVIA centaur (Bayer Diagnostics, Tarrytown, NY, USA). Undifferentiated MSCs were used as a control group.
Animal models were anaesthetised by light ether on the fifth day after STZ injection. An abdominal incision was made parallel to the right costal margin to expose the liver. The UCBMSCs were injected into the liver of GIII animals using a fine needle syringe (dose 2 × 106 cells/injection) while the animals in the GI and GII groups underwent sham operations.(12)
Prior to transplantation of the cell, cultured cells were infused with ferumoxides injectable solution (Bayer Healthcare Pharmaceuticals Inc, Wayne, NJ, USA) that was tracked after intrahepatic transplantation using Prussian blue stain.(13) Estimation of blood glucose levels were done every week after stem cell transplantation for ten weeks.
At every point of time, the animals were anaesthetised with ether and intracardially perfused with 200 mL of freshly prepared ice-cold formaldehyde 4% in phosphate-buffered saline (pH 7.4). The liver and pancreas were dissected out, fixed in 10% neutral buffered formalin (24 hours), dehydrated and paraffin fixed. Sections of 6-µ thickness were prepared and stained with haematoxylin and eosin, Prussian blue and anti-human insulin antibody immunostain (Anti-Human Insulin R/CD220, Biotin, Polyclonal, R&D Systems™, Canada).
Statistical analysis was done using the arithmetic mean, standard deviation, analysis of variance and t-test. All statistical analyses were done using IBM SPSS Statistics version 22 (IBM Corp, Armonk, NY, USA). A p-value ≤ 0.05 was considered to be statistically significant.
RESULTS
The viability of cells was confirmed by trypan blue stain. The living cells did not take up any stain while dead cells were stained blue after isolation of the mononuclear cells and following culture (
Fig. 1
Photomicrograph shows morphological changes of expanded undifferentiated umbilical cord blood mesenchymal stem cells. Living cells did not take up any stain (white arrow) while dead cells were stained blue (black arrow) (Trypan blue × 400).
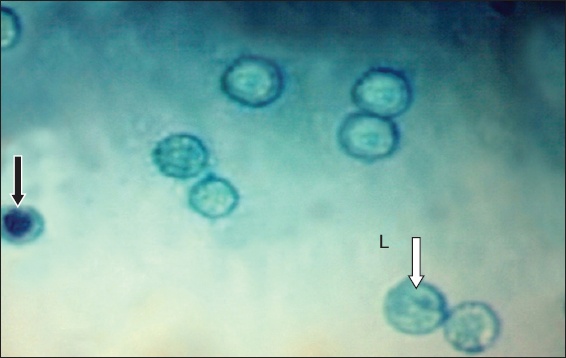
Where the morphological changes of undifferentiated UCBMSCs (1–14 days after culture) were concerned, examination of the primary culture of UCBMSCs (Day 1) revealed the presence of rounded overcrowded cells. These cells were variable in shape and size (
Fig. 2
Photomicrographs show morphological changes in undifferentiated UCBMSCs upon culturing. (a) Cells of variable shape and size were seen on Day 1 of culture (culture media × 200); and (b) evidence of some cells changing their shape and starting to form processes (arrows) was noticed on Day 3 of culture subsequent to removal of supernatant and changing of media (culture media × 100).
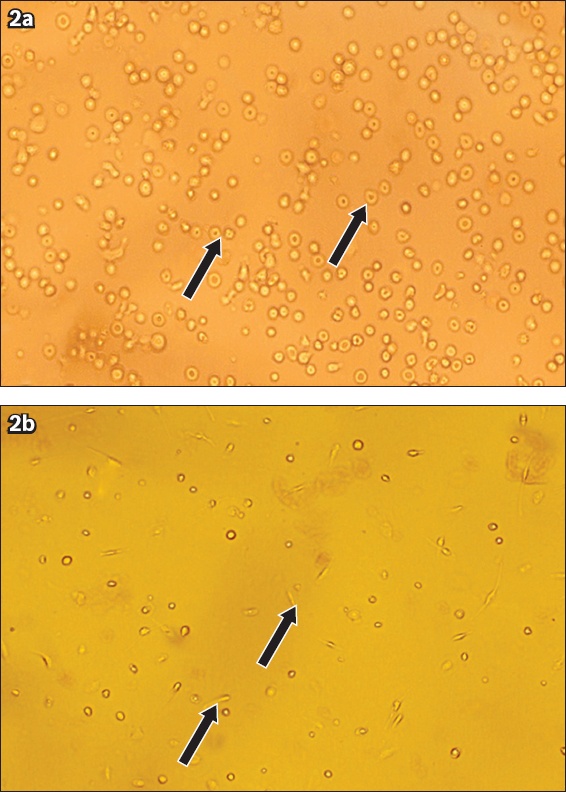
Fig. 3
Photomicrograph shows morphological changes in umbilical cord blood mesenchymal stem cells that differentiated into islet-like clusters (arrows) on Day 21 (culture media × 100).
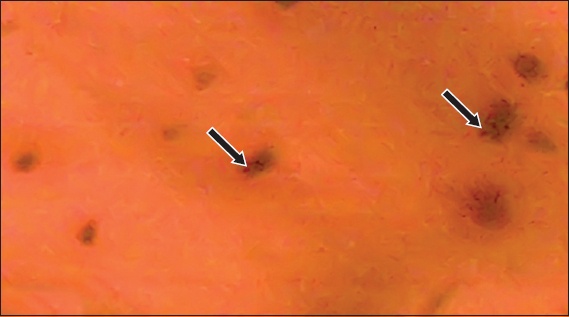
Fig. 4
Gel electrophoresis of RT-PCR products for detection of insulin gene expression. (a) β-actin gene (lanes 1, 2, 3, 4 and 5), which was 160 bp in length, was the positive control and indicated an intact experimental system; (b) insulin gene (Lanes 1, 2, 3, 4 and 5), which was 263 bp in length, showed positive insulin expression in differentiated UCBMSCs in Lanes 1 and 4, negative insulin expression in undifferentiated UCBMSCs in Lanes 2 and 3, and a negative control in lane 5. Lane M was 50-bp DNA marker. RT-PCR: reverse transcription polymerase chain reaction; UCBMSCs: umbilical cord blood mesenchymal stem cells
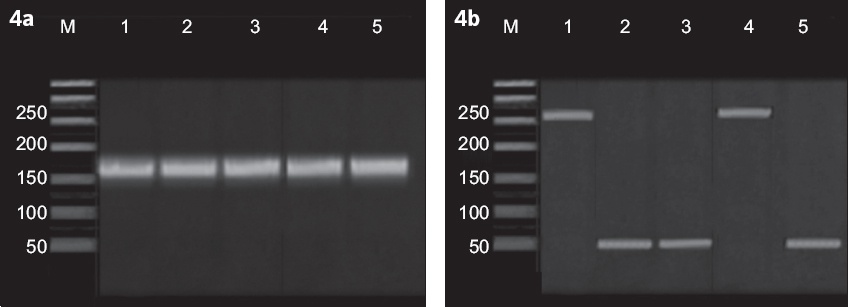
Fig. 5
Graphs show analysis of insulin expression by flow cytometric analysis showed that (a) only 1.1% of undifferentiated UCBMSCs were insulin-positive cells while (b) 40% of differentiated UCBMSCs were insulin positive or expressing insulin. APC: allophycocyanin; UCBMSCs: umbilical cord blood mesenchymal stem cells
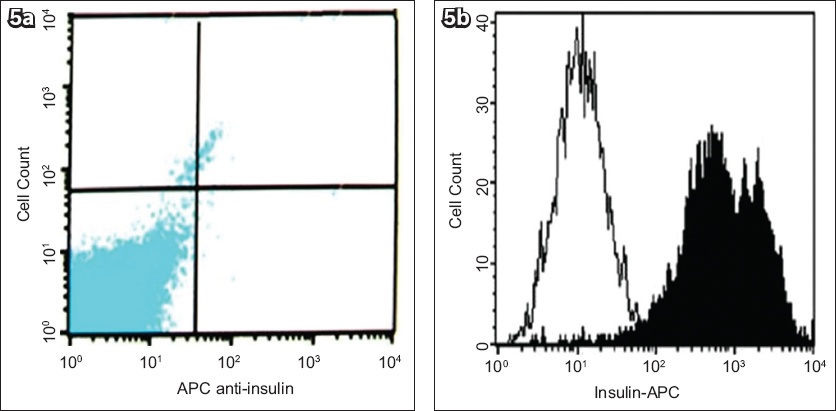
Fig. 6
Graph shows mean cytoplasmic insulin expression of undifferentiated and differentiated MSCs by flow cytometry. MSCs: umbilical cord blood mesenchymal stem cells
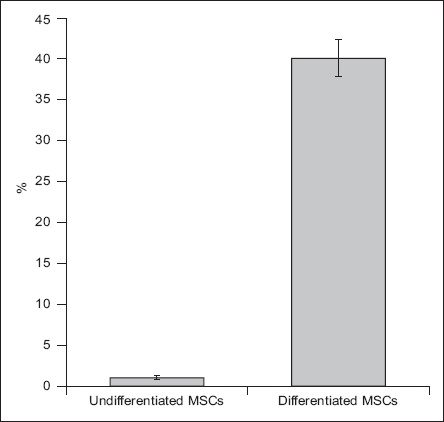
Fig. 7
Graph shows mean insulin concentrations of undifferentiated and differentiated MSCs by insulin detection assay. MSCs: umbilical cord blood mesenchymal stem cells
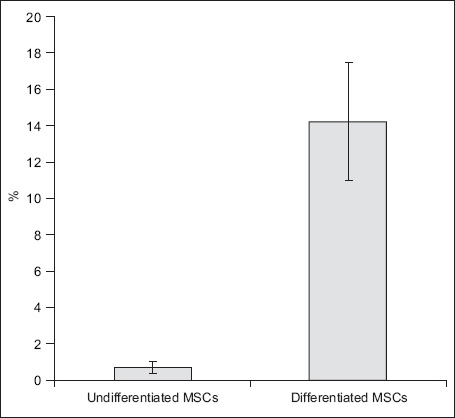
During in vivo studies, the biochemical assay showed that the mean fasting blood glucose level of the control group was 91.95 mg/dL (
Fig. 8
Chart shows mean fasting blood glucose levels from Day 0 to Week 10 after transplantation in control rats, rats with diabetes mellitus without UCBMSC transplantation (diabetic group) and rats with diabetes mellitus with UCBMSC transplantation (MSC group). UCBMSC: umbilical cord blood mesenchymal stem cell
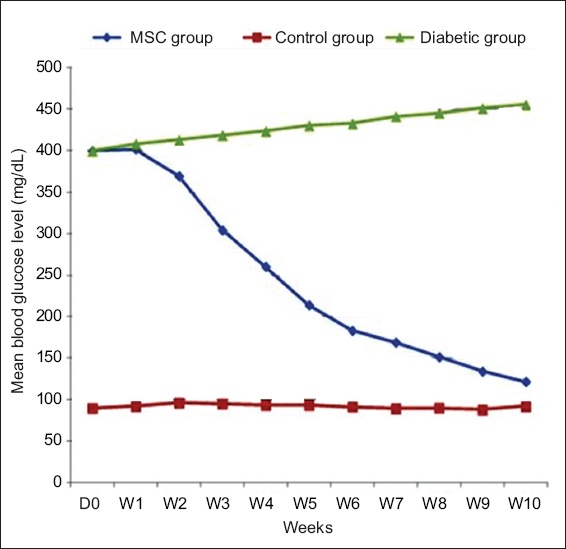
During histological, histochemical and immunohistochemical studies, haematoxylin and eosin-stained sections showed that, in both control (GI rats) and animals with diabetes mellitus without stem cells transplantation (GII rats), the liver showed normal liver architecture (
Fig. 9
Photomicrographs show histological analysis of rat liver sections. (a) Rats with diabetes mellitus without UCBMSC transplantation had normal arrangement of hepatocytes (arrows) around portal triad (*) (Haematoxylin & eosin × 400). (b) Rats with diabetes mellitus with UCBMSC transplantation had intact cell clusters within liver parenchyma that appeared larger than hepatocytes (arrows) (Haematoxylin & eosin × 1,000). (c) Rats with diabetes mellitus with UCBMSC transplantation had intact cell clusters around central vein that appeared larger than hepatocytes (arrows) (Haematoxylin & eosin × 1,000). CV: central vein; UCBMSC: umbilical cord blood mesenchymal stem cell
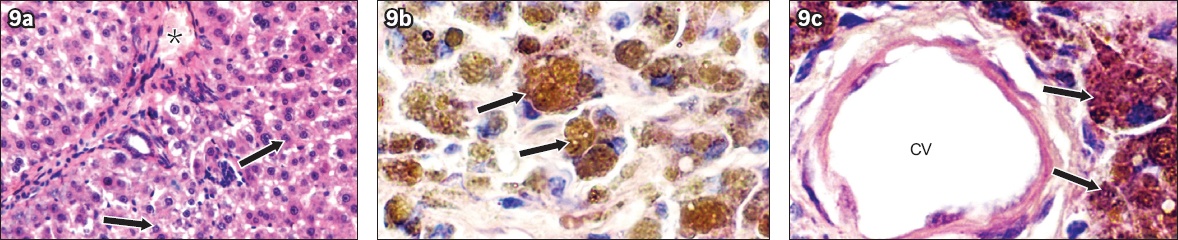
Fig. 10
Photomicrographs show histochemical analysis of rat liver sections. (a) Rats with diabetes mellitus without UCBMSC transplantation had few scattered positive bright blue staining mainly in the Kupffer cells (arrows) (Prussian blue with counter nuclear fast red × 1,000). (b) Rats with diabetes mellitus with UCBMSC transplantation had cell clusters with bright blue staining cytoplasm (arrows) (Prussian blue with counter nuclear fast red × 1,000). (c) Rats with diabetes mellitus with UCBMSC transplantation had cell clusters with bright blue staining cytoplasm around the central vein (arrows) (Prussian blue with counter nuclear fast red × 40). CV: central vein; UCBMSC: umbilical cord blood mesenchymal stem cell

Fig. 11
Photomicrographs show immunohistochemical analysis of rat liver sections. (a) Control rats had normal arrangement of hepatocytes (arrow) around the central vein, with negative anti-human insulin immunostaining (Anti-human insulin immunostain × 400). (b) Rats with diabetes mellitus without UCBMSC transplantation had normal arrangement of hepatocytes (arrows) around the central vein (CV), with negative anti-human insulin immunostaining (Anti-human insulin immunostain × 400). (c) Rats with diabetes mellitus with UCBMSC transplantation (arrows) had positive anti-human insulin immunostaining (Anti-human insulin immunostain × 400). (d) Rats with diabetes mellitus with UCBMSC transplantation had positive anti-human insulin immunostaining around the central vein (cells stained brown indicated with arrows: transplanted stem cells) (Anti-human insulin immunostain × 400). CV: central vein; UCBMSC: umbilical cord blood mesenchymal stem cell
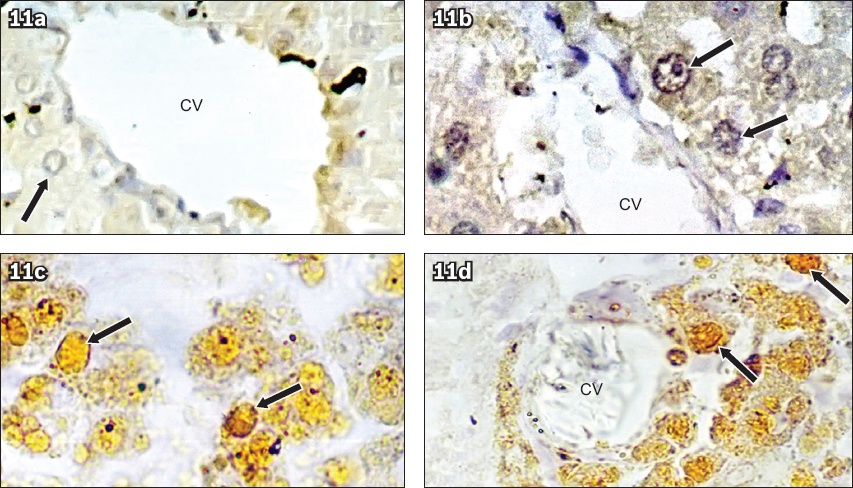
DISCUSSION
In vitro studies on MSCs derived from the human bone marrow show that when subjected to appropriate genetic/microenvironmental manipulation, they express genes and transcription factors similar to that of the endocrine pancreas.(14-16) Other researchers have contradicted the above studies, arguing that MSCs obtained from the bone marrow could not transdifferentiate into fully functional β-cells.(17-19) MSCs derived from human adipocytes were induced in vitro to differentiate into functional β-cells that could secrete insulin, hence reducing the blood glucose levels in induced rats with diabetes mellitus.(20,21) In our study, the UCBMSCs were preferred over the other stem cell types due to their unique advantages, such as painless collection procedure, easy availability, high efficiency of differentiation and faster self-renewal, with lower risk for graft versus host disease.(22) Studies by Sun et al(23) and Jing et al(24) showed that these cells, when transplanted into normal rat tissues, failed to produce tumours or any immune rejection response. Moreover, reports on the efficiency of UCBMSCs for the treatment of various injuries and diseases, both clinically and pre-clinically, have been documented in the recent past.(25,26)
Various complex procedures and strategies have been used for inducing the UCBMSCs to differentiate into insulin-producing cells.(27,28) Since high glucose is considered as a powerful inducing agent for the differentiation of islet cells,(29) the UCBMSCs in the present study were initially induced in vitro by using high glucose medium to produce small aggregates of cells that exhibited positive insulin gene expression confirmed by RT-PCR. Based on the studies by Li et al(30) that have emphasised the beneficial role of Exendin-4 in the β-cell differentiation and inhibition of β-cell apoptosis, the cells in our study were subsequently supplemented with Exendin-4. The differentiation of insulin-producing cells from UCBMSCs was confirmed using flow cytometric analysis. Our results illustrated that ~40% of differentiated UCBMSCs were insulin positive. Moreover, the cells remained negative for CD34 and positive for CD44. The results of the present study indicate a 40% induction of UCBMSCs into insulin-producing cells when compared to previously reported data,(31) wherein only 5%–15% of insulin-producing cells were derived from UCBMSCs. Moreover, an insulin detection test also depicted high total protein content in the insulin-producing cells when compared to undifferentiated cells.
In the present study, after the transplantation of insulin-producing cells in the rats, the blood sugar levels of STZ-induced animals dropped considerably from 399 mg/dL to 121.9 mg/dL over a period of five weeks and remained stable during the subsequent five weeks of observation. However, the animals of the untreated group induced with STZ remained hyperglycaemic. The present study also showed that although blood glucose levels were reduced in rats with diabetes mellitus that received transplanted differentiated UCBMSCs, it was still higher compared to the control group.
The histological confirmation proved by haematoxylin and eosin staining showed intact cell clusters larger than hepatocytes situated within the liver parenchyma and around the central veins in rats that received transplantation of differentiated UCBMSCs. Likewise, Prussian blue staining was also positive, presenting cell clusters with bright blue staining cytoplasm in animals of the same group, signifying that these labelled cells were not resident macrophages with engulfed iron particles. More importantly, anti-human insulin immune-stained sections showed no expression of human insulin in the liver parenchyma, both for control rats and animals with diabetes mellitus without stem cell transplantation. On the contrary, clusters of positively stained cells with fine brownish granules within their cytoplasm were seen between hepatocytes and around the central veins of the liver lobules in animals that received transplanted differentiated UCBMSCs.
Taken together, all these findings strongly suggest the possible role of the transplanted UCBMSCs in decreasing and alleviating the levels of blood glucose in hyperglycaemic STZ-induced rats. The current study also confirmed the findings of previous studies while, when cultured under specific conditions, UCBMSCs could differentiate into insulin-producing cells.(32) With a number of valid scientific studies related to the effectiveness, safety and possibility of the differentiation of UCBMSCs into insulin-producing cells, a further step towards clinical trials is anticipated to determine the feasibility of these cell therapies. The only challenge would be to harvest sufficient numbers of functional islet pancreatic cells that can remain active in vivo.