A 35-year-old man who was admitted to the intensive care unit for dengue fever developed hypotension on Day 2 of hospitalisation. He had no significant past medical history. His height was 1.7 m and weight was 70 kg. In the past 24 hours, he had received 3 L of intravenous normal saline but had passed urine only twice. He was alert and there was no obvious blood loss. His vital signs were as follows: temperature 37°C, pulse rate 120/min, respiratory rate 20/min, blood pressure (by oscillometry in both arms) 80/60 mmHg. Capillary refill time was six seconds, and venous lactate was 5 mmol/L.
IDENTIFICATION OF SHOCK
The patient had circulatory shock, marked by a delayed capillary refill time of six seconds (normal ≤ 3 seconds).(1) Circulatory shock is a state of low tissue oxygenation (tissue hypoxia) owing to oxygen supply-demand mismatch (i.e. oxygen supply is insufficient for end-organ demands) or impaired oxygen utilisation (e.g. due to mitochondrial dysfunction). Persistent circulatory shock will eventually lead to end-organ damage. The patient also had hypotension, which is defined as a systolic blood pressure < 90 mmHg(2) or a mean arterial pressure < 65 mmHg.(1) Mean arterial pressure is, in turn, defined as the sum of one-third of the systolic blood pressure and two-thirds of the diastolic blood pressure.
Oxygen supply is predominantly determined by cardiac output, haemoglobin concentration and arterial blood oxygen saturation. Blood pressure is determined by both cardiac output and systemic vascular resistance. Given this dual dependency for blood pressure, low blood pressure can occur even in patients with high cardiac output if systemic vascular resistance is very low. Low cardiac output can affect both oxygen supply and blood pressure, and circulatory shock and hypotension can co-exist. However, discordant situations can occur. Circulatory shock can occur in the absence of hypotension (i.e. occult hypoperfusion) if cardiac output is low and systemic vascular resistance is very high. Hypotension can also occur in the absence of circulatory shock if hypotension is solely due to low systemic vascular resistance (i.e. cardiac output is preserved), or if the impact of low cardiac output is counterbalanced by high haemoglobin concentration, high oxygen saturation and low end-organ demand.
GENERAL APPROACH TO SHOCK MANAGEMENT
The approach to shock management (
Fig. 1
Diagram shows circulatory shock management. *If ScvO2 and PcvCO2 measurements are not available, then one should at least meet the conventional targets for blood pressure (mean arterial pressure 65 mmHg or systolic blood pressure 90 mmHg), haemoglobin (7–9 g/dL) and SaO2 (94%–98%). CO2: carbon dioxide; PaCO2: arterial carbon dioxide partial pressure; PcvCO2: central venous carbon dioxide partial pressure; SaO2: arterial oxygen saturation; ScvO2: central venous oxygen saturation
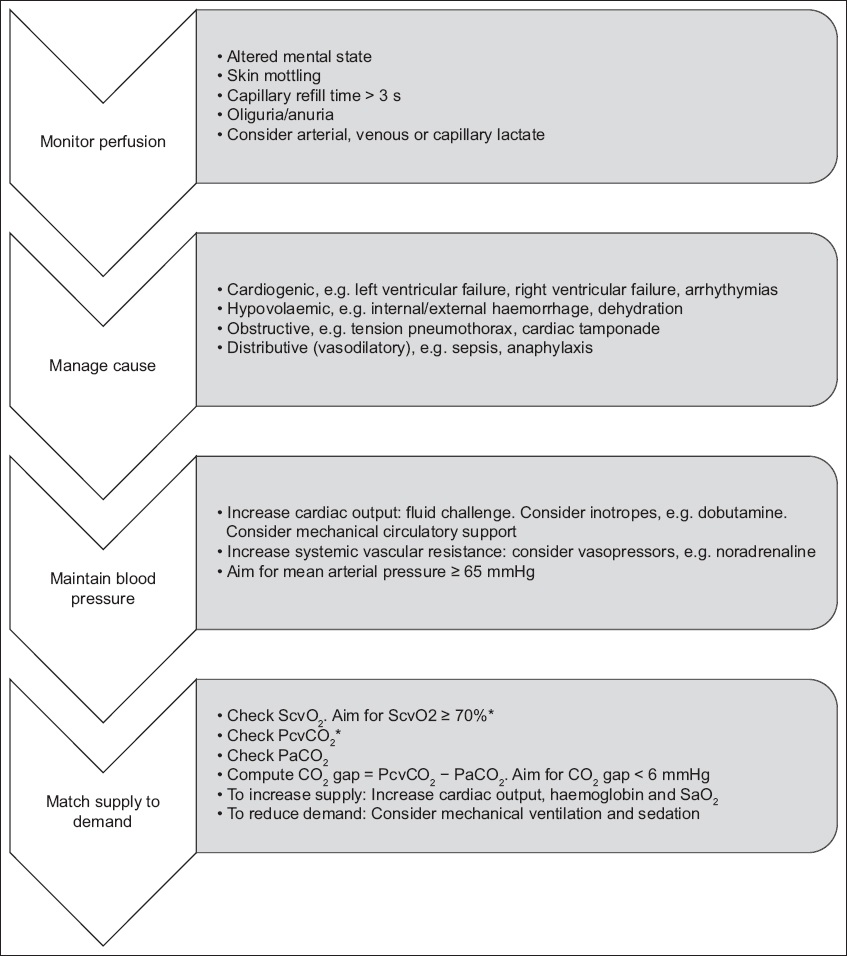
Table I
Methods to assess perfusion.
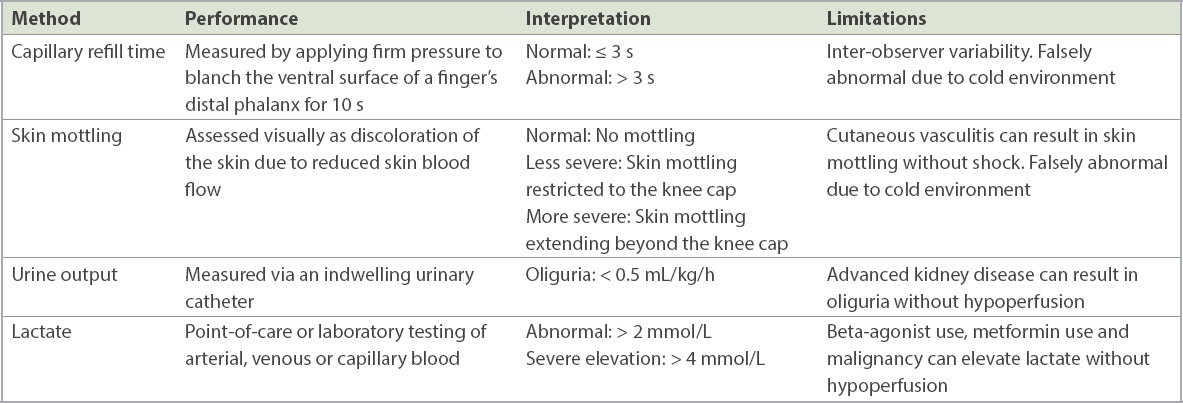
The second step for shock management involves uncovering and treating the cause of circulatory shock. The same aetiological framework also applies to hypotension. Four broad pathophysiological causes may be present, namely cardiogenic, hypovolaemic, obstructive and distributive (vasodilatory).(2) These causes can coexist, such as in sepsis, where septic cardiomyopathy, dehydration and vasodilatation can all contribute to shock. It might be difficult to assess the aetiology based on clinical examination alone. At the bedside, history-taking and physical examination should be supplemented by point-of-care echocardiography and lung ultrasonography (
Table II
Causes of circulatory shock or hypotension.
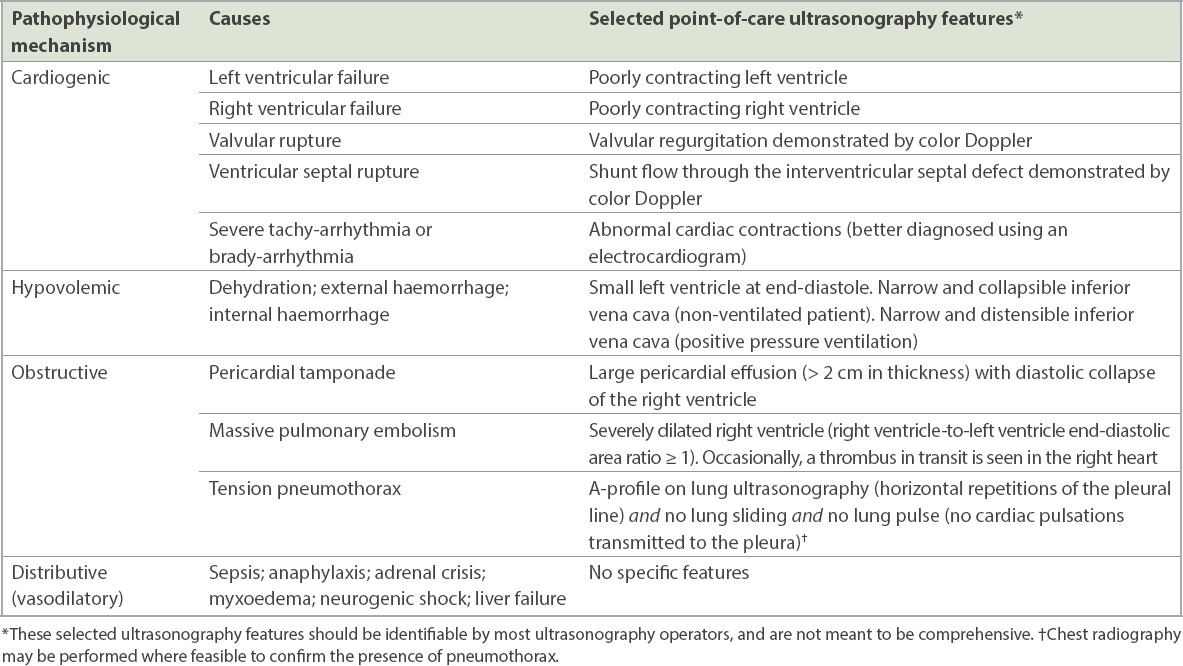
The third step for shock management involves maintaining adequate blood pressure. A systolic blood pressure of at least 90 mmHg(2) and a mean arterial pressure of at least 65 mmHg should be targeted.(1) Blood pressure can be measured in a non-invasive or invasive manner (Key Clinical Tool 1). To boost blood pressure, fluids should be given to patients who are fluid responsive (i.e. stroke volume should increase by at least 10% when a fluid bolus is given). Stroke volume needs to be measured accurately using either transthoracic echocardiography (measuring the left ventricular outflow tract velocity-time integral) or thermodilution methods (e.g. using a pulmonary artery catheter). If stroke volume cannot be measured, a surrogate measure may be pulse pressure (difference between systolic blood pressure and diastolic blood pressure).(5)
The probability of being fluid responsive can be tested by relying on cardiopulmonary interaction (e.g. inferior vena cava variability, pulse pressure variation and stroke volume variation) or by using functional haemodynamic tests (e.g. end-expiratory occlusion, passive leg raising). However, all of these methods are imperfect and may not be available outside of intensive care units.(6) If fluid responsiveness cannot be assessed with confidence, a fluid challenge should be performed, using a fast bolus of a crystalloid solution (e.g. 500 mL of normal saline for < 30 minutes).(7) The fast fluid infusion rate can be assured if fluid is administered using an infusion pump; for example, setting the pump at 1,200 mL/h would mean that 500 mL of fluid will be given over 25 minutes. After volume expansion has been attempted, continued hypotension should be treated with a vasopressor (e.g. noradrenaline). If cardiac contractility is known to be low, inotropes (e.g. dobutamine) can be considered. Early use of vasopressors and inotropes can be facilitated by peripheral administration before a central venous catheter is available.(8) At the earliest opportunity, a central venous line should be inserted for further monitoring and resuscitation.
The final step for shock management involves matching oxygen supply to end-organ demand. This step requires a central venous catheter or peripherally inserted central catheter with its tip around the junction of the superior vena cava and the right atrium. In this position, blood drawn from the catheter would be the ‘central venous’ blood; blood gas analysis would yield the central venous oxygen saturation (ScvO2) and the central venous carbon dioxide partial pressure (PcvCO2). Paired assay of arterial blood would provide the arterial carbon dioxide partial pressure (PaCO2) and CO2 gap (Key Clinical Tool 2). In general, oxygen supply can be improved via increases in stroke volume (via volume expansion in fluid-responsive patients and inotropes in patients with low cardiac contractility), haemoglobin and arterial oxygen saturation (SaO2). Oxygen demand can be reduced via mechanical ventilation and sedation. If ScvO2 and PcvCO2 measurements are not available, then the conventional targets for blood pressure (mean arterial pressure 65 mmHg or systolic blood pressure 90 mmHg), haemoglobin (7–9 g/dL)(9) and SaO2 (94%–98%) should at least be met.(10) Mechanical circulatory support (e.g. veno-arterial extracorporeal membrane oxygenation) may be required as a last resort for refractory shock.
Key clinical tool 1: blood pressure measurement
Blood pressure should be measured using a sphygmomanometer or an oscillometric device, using an appropriately sized cuff. This cuff is most commonly applied at the brachial artery, although ankle or thigh blood pressure measurements will be necessary if satisfactory cuff placement is not possible in the arms.(11) For accurate and continuous blood pressure monitoring in critically ill patients, invasive blood pressure measured via an arterial line is the reference standard. Although the more distal positions of arterial line sites give higher systolic blood pressure readings, the mean arterial pressure is similar across sites and is the preferred metric for blood pressure evaluation.
For accurate invasive blood pressure measurement, care should be taken with regard to three technical details.(12) Firstly, the arterial line must be zeroed by transiently opening the line to atmospheric pressure and pressing the ‘zero’ button on the monitoring device. Secondly, the arterial line must be levelled by placing the transducer at the level of the aortic root (i.e. phlebostatic axis), which is marked by the intersection of the fourth intercostal space and the mid-axillary line. Thirdly, the arterial line must be appropriately damped, which can be checked by performing a saline flush and looking for 1–2 oscillations of the arterial line waveform after the fast flush. If no oscillations are present, the arterial line is over-damped and any line obstruction by air bubbles, clots and kinks must be fixed. If > 2 oscillations are present, the arterial line is under-damped (e.g. due to stiff non-compliant tubing), leading to falsely high systolic blood pressure and falsely low diastolic blood pressure, although the mean arterial pressure will remain accurate.
Key clinical tool 2: ScvO2 and CO2 gap
These clinical tools are key for assessing oxygen supply and demand.(13) When end-organs undergo aerobic metabolism, oxygen is taken up from blood and CO2 is released into blood. Venous blood returning to the heart would then have lower oxygen content and higher CO2 content compared to that of the arterial blood being supplied to the end-organs. Venous blood returning to the heart is assayed around the junction of the superior vena cava and the right atrium, via central venous catheters or peripherally inserted central catheters, allowing measurements of ScvO2 and PcvCO2. Arterial blood can be simultaneously sampled via an invasive arterial cannula or via intermittent arterial blood draws, allowing measurement of arterial PaCO2.
High demand compared to supply would render the ScvO2 lower than 70%. When such a patient has circulatory shock, efforts to increase supply or reduce demand are warranted. A low demand compared to supply would mean that the ScvO2 would be 70% or higher. When such a patient has circulatory shock, two possibilities could exist. Firstly, oxygen demand is low owing to damaged tissue metabolism, which is, in turn, due to mitochondrial dysfunction (e.g. sepsis, cyanide poisoning), necessitating treatment of the underlying cause. Secondly, oxygen demand is low owing to reduced oxygen extraction by ischaemic tissue in the setting of poor blood flow into end-organs, which can be reversed by improving cardiac output. Differentiating these two possibilities requires the CO2 gap, which is the difference between PcvCO2 and PaCO2. The CO2 gap would be 6 mmHg or less if tissue metabolism is damaged, while it would be > 6 mmHg if tissue metabolism is preserved within ischaemic tissues. The widened CO2 gap in the context of poor blood flow into end-organs is due to continued diffusion of CO2, which has higher blood solubility than O2, from ischaemic tissues into the venous blood.
Table III
Oxygen supply and demand analysis in shock.

TAKE HOME MESSAGES
- Circulatory shock is the state of low tissue oxygenation (tissue hypoxia) due to oxygen supply-demand mismatch (i.e. oxygen supply is insufficient for end-organ demands) or impaired oxygen utilisation (e.g. due to mitochondrial dysfunction).
- Hypotension is defined as a systolic blood pressure < 90 mmHg or a mean arterial pressure < 65 mmHg. Circulatory shock can occur in the absence of hypotension (i.e. occult hypoperfusion).
- Oxygen supply is predominantly determined by cardiac output, haemoglobin concentration and arterial blood oxygen saturation. Blood pressure is determined by cardiac output and systemic vascular resistance.
- The approach to shock management comprises four general steps: monitor perfusion, manage cause, maintain blood pressure and match supply to demand.
- Four broad pathophysiological causes may be present and may coexist: cardiogenic, hypovolaemic, obstructive and distributive (vasodilatory).
Given the presence of circulatory shock, the patient underwent a thorough physical examination, which revealed cool peripheries and mild peripheral oedema. Point-of-care echocardiography using a handheld device demonstrated poor biventricular contraction and no large pericardial effusion. A fluid challenge with 500 mL of normal saline over 25 minutes did not improve blood pressure. Low-dose noradrenaline was started, targeting a mean arterial pressure of 65 mmHg. The ScvO2 was 60% despite an oxygen saturation of 96% on pulse oximetry and a haemoglobin level of 12 g/dL. Troponin I was elevated, and an electrocardiogram demonstrated sinus tachycardia and nonspecific ST/T-wave changes. An urgent bedside echocardiogram by the on-call cardiologist showed a left ventricular ejection fraction of 15%, suggesting dengue with cardiac involvement. Low-dose dobutamine was administered intravenously at 5 mcg/kg/min, after which the ScvO2 increased to 70% and the CO2 gap was 5 mmHg. The patient was transferred from the medical intensive care unit (ICU) to the cardiothoracic ICU for potential institution of mechanical circulatory support. Fortunately, with careful titration of fluids and dobutamine, he did not require mechanical ventilation or extracorporeal membrane oxygenation. His perfusion and left ventricular function gradually improved over the next five days, allowing him to be weaned off dobutamine.